Sustainable livestock farming: Progress since 1950
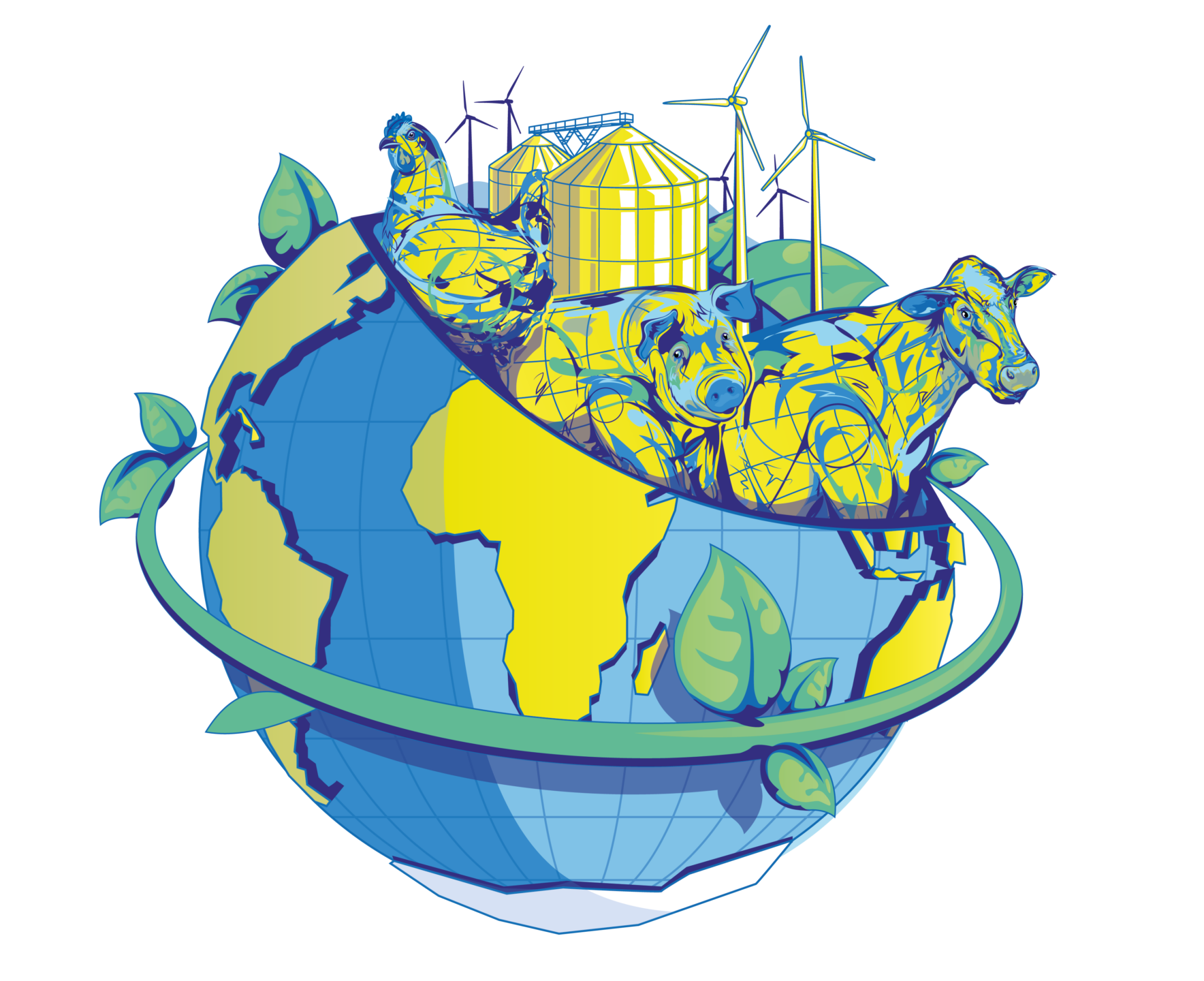
by Ilinca Anghelescu, Global Director Marketing Communications, EW Nutrition
Summary
- Global GHG Emissions and Agriculture:
- Agriculture-related emissions account for 31% of global anthropogenic emissions, with a growing share coming from food-related activities outside traditional farming, such as processing and transportation.
- This represents a significant decrease from the 1950s when agriculture contributed to 58% of global emissions, a decrease largely due to the increased use of fossil fuels.
- Population Growth and Emissions:
- The global population has increased by 220% since 1950, leading to a threefold increase in agri-food emissions, now totaling 9-10 billion metric tons of CO2-equivalent annually.
- Meat Production Growth:
- Meat production has seen a 690% increase since 1950, driven by population growth, economic development, urbanization, technological advancements, and intensification of livestock production.
- Technological improvements have significantly increased livestock yield, including higher carcass weights, improved feed efficiency, and greater output per animal.
- Feed Conversion Ratio (FCR) as a Sustainability Metric:
- FCR, which measures the efficiency of feed conversion into body mass, has improved dramatically for poultry, pigs, and cattle since the 1950s.
- Improved FCR contributes to more efficient resource use, reduced environmental impact, better animal welfare, and economic viability.
- Livestock Emissions and Land Use:
- Livestock-related emissions have increased by 14% since 2000, with a significant portion coming from enteric fermentation in ruminants and land use changes.
- Pasture and grazing land have expanded slightly, while the land for feed crops has increased substantially due to intensified livestock production.
- Food Loss and Its Impact on Sustainability:
- Food loss, occurring primarily before the consumer stage, remains stable at around 13%. Reducing food loss is critical for improving food security, economic efficiency, and minimizing the environmental footprint of livestock production.
- Future Strategies for Sustainability:
- The article emphasizes the need for ongoing investment in technology, optimization of feed efficiency, sustainable land use, and improved methods for tracking and reducing emissions to ensure the future sustainability of livestock farming.
As the global demand for animal products continues to rise, so do various claims about the impact of agriculture on greenhouse gas emissions. A study commissioned by the United Nations’ Food and Agriculture Organization (FAO) concluded that, according to the most recent data, agri-food system emissions totaled 16.5 billion metric tons of CO2 equivalent, representing 31% of global anthropogenic emissions.
Of these 31%, the most important trend highlighted by FAO was the “increasingly important role of food-related emissions generated outside of agricultural land, in pre- and post-production processes along food supply chains”. The food supply chain (food processing, packaging, transport, household consumption and waste disposal) is thus set to become the top GHG emitter, above farming and land use.
How bad is 31%?
While 31% is a large figure, even this estimate represents a significant decrease from the 1950s, when agri-food emissions constituted approximately 58% of total anthropogenic emissions: “From 1850 until around 1950, anthropogenic CO2 emissions were mainly (>50%) from land use, land-use change and forestry”, states the latest IPCC report.
Figure 1. Source: IPCC AR6 Report, 2023. LULUCF = Land Use, Land-Use Change and Forestry
As the IPCC graph in Figure 1 indicates, the percentage decrease is mostly due to the rising prevalence of oil and coal in CO2 emissions over the recent decades, as shown in Figure 2 below.
Annual greenhouse gas (GHG) emissions worldwide from 1990 to 2022, by sector (in million metric tons of carbon dioxide equivalent)
Figure 2. Source: Statista
Total population and agri-food emission changes, 1950 – today
The global population increased by approximately 220%, from 2.5 billion in 1950 to 8 billion in 2023. In the meantime, estimates suggest that, in the 1950s, agri-food systems were responsible for approximately 2-3 billion metric tons of CO2-equivalent (CO2e) emissions per year. This figure includes emissions from livestock, rice paddies, fertilizer use, and land-use change (e.g., deforestation for agriculture).
Assessments generally agree that today’s agri-food systems contribute approximately 9-10 billion metric tons of CO2e annually, a threefold increase from 1950. This includes emissions from agriculture (e.g., livestock, crop production), food processing, transportation, and land-use changes.
This increase is consistent with FAO’s new findings, of food chain climbing to the top of agri-food emitters.
But where did these increased emissions come from?
A look at the graph below gives us an indication: world poverty rate decreased massively between 1950 and today. While COVID brought a setback, the historical data would clearly indicate a correlation between the increased output in agri-food systems and the decreased rate of poverty.
How did poverty rates decline so steeply? The reasons lie, to a large extent, in technological innovation, especially in genetics and farm management, and in the increased apport of plentiful and affordable meat protein to the world. The numbers below build an image of an industry that produces better, more, and cheaper.
Global meat production: 1950 vs. Present
Then…
In 1950, the estimated total meat production was of approximately 45 million metric tons.
Key Producers: The United States, Europe, and the Soviet Union were the primary producers of meat.
Types of Meat: Production was largely dominated by beef and pork, with poultry being less significant.
…and now
Now, the total meat production lies somewhere around 357 million metric tons (as of recent data from FAO)., representing a 53% increase from 2000 and a staggering 690% increase from 1950.
Key Producers: Major producers include China, the United States, Brazil, and the European Union.
Types of Meat: Significant increases in poultry production, with pork remaining a leading source of meat, especially in Asia. Beef production has also increased, but at a slower rate than poultry and pork.
Factors contributing to increased meat production
Population Growth: The world population has grown from approximately 2.5 billion in 1950 to over 8 billion today, driving increased demand for meat.
Economic Growth and Urbanization: Rising incomes and urbanization have led to shifts in economic power and dietary preferences, with more people consuming higher quantities of meat, especially in developing countries.
Technological Advancements: Improvements in animal breeding, feed efficiency, and production systems have increased the efficiency and output of meat production.
Intensification of Livestock Production: The shift from extensive to intensive livestock production systems has allowed for higher meat yields per animal.
Global Trade: Expansion of global trade in meat and meat products has facilitated the growth of production in countries with comparative advantages in livestock farming.
Livestock yield increase, 1950 to the present
The increase in livestock yield for cattle, pigs, and chickens between 1950 and the present has been significant due to advances in breeding, nutrition, management practices, and technology.
Beef
1950s
- Average Carcass Weight: In the 1950s, the average carcass weight of beef cattle was about 200 to 250 kilograms (440 to 550 pounds).
- Dressing Percentage: The dressing percentage (the proportion of live weight that becomes carcass) was typically around 50-55%.
Present Day
- Average Carcass Weight: Today, the average carcass weight of beef cattle is approximately 300 to 400 kilograms (660 to 880 pounds).
- Dressing Percentage: The dressing percentage has improved to about 60-65%.
Increase in Beef Cattle Yield
- Increase in Carcass Weight: The average carcass weight has increased by about 100 to 150 kilograms (220 to 330 pounds) per animal.
- Improved Dressing Percentage: The dressing percentage has increased by about 5-10 percentage points, meaning a greater proportion of the live weight is converted into meat.
Dairy
1950s
- Average Milk Yield per Cow: Approximately 2,000 to 3,000 liters per year, depending on the region.
Present Day
- Average Milk Yield per Cow: Approximately 8,000 to 10,000 liters per year globally, with some countries like the United States achieving even higher averages of 10,000 to 12,000 liters per year.
Increase in Milk Yield:: Milk yield per cow has increased about 4-5 times due to genetic selection, improved nutrition, technological advancements, and better herd management.
Chickens (Layers)
1950s
- Average Egg Production per Hen: In the 1950s, a typical laying hen produced about 150 to 200 eggs per year.
Present Day
- Average Egg Production per Hen: Today, a typical laying hen produces approximately 280 to 320 eggs per year, with some high-performing breeds producing even more.
Increase in Egg Yield: The average egg production per hen has increased by approximately 130 to 170 eggs per year.
Chickens (Broilers)
1950s
- Average Yield per Bird: In the 1950s, broiler chickens typically reached a market weight of about 1.5 to 2 kilograms (3.3 to 4.4 pounds) over a growth period of 10 to 12 weeks.
Present Day
- Average Yield per Bird: Today, broiler chickens reach a market weight of about 2.5 to 3 kilograms (5.5 to 6.6 pounds) in just 5 to 7 weeks.
Increase in Yield: The average weight of a broiler chicken has increased by approximately 1 to 1.5 kilograms (2.2 to 3.3 pounds) per bird. Additionally, the time to reach market weight has been nearly halved.
Factors contributing to yield increases
Genetic Improvement:
- Selective Breeding: Focused breeding programs have developed chicken strains with rapid growth rates and high feed efficiency, significantly increasing meat yield.
Nutrition:
- Optimized Feed: Advances in poultry nutrition have led to feed formulations that promote faster growth and better health, using balanced diets rich in energy, protein, and essential nutrients.
Management Practices:
- Housing and Environment: Improved housing conditions, including temperature and humidity control, have reduced stress and disease, enhancing growth rates.
Technological Advancements:
- Automation: Automation in feeding, watering, and waste management has improved efficiency and bird health.
- Health Monitoring: Advances in health monitoring and veterinary care have reduced mortality rates and supported faster growth.
Feed Conversion Efficiency:
- Improved Feed Conversion Ratios (FCR): The amount of feed required to produce a unit of meat has decreased significantly, making production more efficient.
Why Feed Conversion Ratio is a sustainability metric
Feed Conversion Ratio (FCR) is a critical metric in livestock production that measures the efficiency with which animals convert feed into body mass. It is expressed as the amount of feed required to produce a unit of meat, milk, or eggs. Advances in nutrition and precision feeding allow producers to tailor diets that optimize FCR, reducing waste and improving nutrient uptake. Also, breeding programs focused on improving FCR can lead to livestock that naturally convert feed more efficiently, supporting long-term sustainability.
Poultry (Broilers): From the 1950s, improved from approximately 4.75 kg/kg to 1.7 kg/kg.
Pigs: From the 1950s, improved from about 4.5 kg/kg to 2.75 kg/kg.
Cattle (Beef): From the 1950s, improved from around 7.5 kg/kg to 6.0 kg/kg.
Figure 4. Evolution of FCR from 1950
FCR is crucial for livestock sustainability for several reasons, as shown below.
1. Resource efficiency
– Feed Costs: Feed is one of the largest operational costs in livestock production. A lower FCR means less feed is needed to produce the same amount of animal product, reducing costs and improving profitability.
– Land Use: Efficient feed conversion reduces the demand for land needed to grow feed crops, helping to preserve natural ecosystems and decrease deforestation pressures.
– Water Use: Producing less feed per unit of animal product reduces the water needed for crop irrigation, which is crucial in regions facing water scarcity.
2. Environmental impact
– Greenhouse Gas Emissions: Livestock production is a significant source of greenhouse gases (GHGs), particularly methane from ruminants and nitrous oxide from manure management. Improved FCR means fewer animals are needed to meet production goals, reducing total emissions.
– Nutrient Runoff: Efficient feed use minimizes excess nutrients that can lead to water pollution through runoff and eutrophication of aquatic ecosystems.
3. Animal welfare
– Health and Growth: Optimizing FCR often involves improving animal health and growth rates, which can lead to better welfare outcomes. Healthy animals grow more efficiently and are less susceptible to disease.
4. Economic viability
– Competitiveness: Lowering FCR improves the economic viability of livestock operations by reducing input costs and increasing competitiveness in the global market.
– Food Security: Efficient livestock systems contribute to food security by maximizing the output of animal protein relative to the input of resources.
Improving FCR is essential for achieving sustainability in livestock production. It leads to more efficient resource use, reduced environmental impact, enhanced economic viability, and supports the well-being of animals. As global demand for animal products continues to rise, optimizing FCR will be crucial in balancing production with the need to protect and preserve natural resources.
FAOSTAT 2023
Livestock emissions
Livestock emissions can be direct (farm-gate) or indirect (land use). Pre- and post-production emissions are considered separately, since they refer to emissions from manufacturing, processing, packaging, transport, retail, household consumption, and waste disposal.
Farm-gate emissions
Global farm-gate emissions (related to the production of crops and livestock) grew by 14% between 2000 and 2021, to 7.8 Gt CO2 eq, see below. 53% come from livestock-related activities, and the emissions from enteric fermentation generated in the digestive system of ruminant livestock were alone responsible for 37 percent of agricultural emissions (FAOSTAT 2023).
Land use for livestock
Land use emissions contribute a large share to agricultural emissions overall, especially through deforestation (~74% of land-use GHG emissions). The numbers have declined in recent years, to a total of 21% reduction between 2000 and 2018.
The other side of the coin is represented by the increased land usage for livestock, either directly for grazing or indirectly for feed crops.
1. Pasture and grazing land
1950: Approximately 3.2 billion hectares (7.9 billion acres) were used as permanent pastures.
Present: The area has increased to around 3.5 billion hectares (8.6 billion acres).
Change: An increase of about 0.3 billion hectares (0.7 billion acres).
2. Land for Feed Crops
1950: The land area dedicated to growing feed crops (such as corn and soy) was significantly less than today due to lower livestock production intensities and smaller scale operations. Feed crops likely accounted for about 200-250 million hectares of the cropland, although figures are evidently difficult to estimate.
Present: Of the approx. 5 billion hectares of land globally used for agriculture, about 1.5 billion hectares are dedicated to cropland.
The increase in cropland hectares is a direct consequence of the intensification of demand for livestock production. To keep these numbers in check, it is essential that producers strive to use as little feed as possible for as much meat yield as possible – and this directly relates to a key metric of the feed additive industry: Feed Conversion Ratio, mentioned above.
The role of food loss in livestock sustainability
The Food and Agriculture Organization (FAO) of the United Nations defines food loss as the decrease in quantity or quality of food resulting from decisions and actions by food suppliers in the chain, excluding retail, food service providers, and consumers. Food loss specifically refers to food that gets spilled, spoiled, or lost before it reaches the consumer stage, primarily taking place during production, post-harvest, processing, and distribution stages.
Food loss is currently estimated to be relatively stable over the last decades, at around 13%.
Key aspects of food loss
- Stages of Food Loss:
- Production: Losses that occur during agricultural production, including damage by pests or diseases and inefficiencies in harvesting techniques.
- Post-Harvest Handling and Storage: Losses that happen due to inadequate storage facilities, poor handling practices, and lack of proper cooling or processing facilities.
- Processing: Losses during the processing stage, which may include inefficient processing techniques, contamination, or mechanical damage.
- Distribution: Losses that occur during transportation and distribution due to poor infrastructure, inadequate packaging, and logistical inefficiencies.
- Quality and Quantity:
- Quality Loss: Refers to the reduction in the quality of food, affecting its nutritional value, taste, or safety, which may not necessarily reduce its quantity.
- Quantity Loss: Refers to the actual reduction in the amount of food available for consumption due to physical losses.
- Exclusions:
- Retail and Consumer Level: Food loss does not include food waste at the retail or consumer levels, which is categorized as food waste. Food waste refers to the discarding of food that is still fit for consumption by retailers or consumers.
Importance of reducing food loss
Every step along the production chain, each action taken to preserve feed, increase yield, ensure stable and high meat quality, can contribute to reducing food loss and ensuring that animal protein production stays sustainable and feeds the world more efficiently.
- Food Security: Reducing food loss can help improve food availability and access, particularly in regions where food scarcity is a concern. Where we thought we were on our way to eradicate world hunger, recent upticks in several regions show us that progress is not a given.
- Economic Efficiency: Minimizing food loss can improve the efficiency and profitability of food supply chains by maximizing the utilization of resources.
- Environmental Impact: Reducing food loss helps to decrease the environmental footprint of food production by lowering greenhouse gas emissions and minimizing land and water use. This is all the more important in regions where world hunger shows signs of going up. Perhaps not by coincidence are these regions some of the most affected by climate change.
By understanding and addressing the causes of food loss, stakeholders across the food supply chain can work towards more sustainable and efficient food systems.
What’s next?
Improving production practices and technology
Investment in research and development of new technologies that enhance livestock production efficiency and reduce environmental impact is vital for the future sustainability of the sector.
India is a good illustration of room to grow. If we look at cow milk alone, India, with a headcount of approximately 61 million animals, has a total milk production that is neck-and-neck with the United States, whose dairy cow headcount is in the neighborhood of 9.3 million. India’s milk yield sits around 1,600 liters/animal/year, compared to the US’s average of 10,700 liters.
Optimizing Feed Efficiency
Continued focus on improving FCR through genetic selection, optimized nutrition, and advanced management practices will be crucial for reducing the environmental footprint of livestock production.
Promoting Sustainable Land Use
Strategies to balance the need for increased livestock production with sustainable land use practices are essential. This includes adopting agroecological approaches and improving the efficiency of feed crop production.
Reducing Food Loss
Stakeholders across the food supply chain must prioritize reducing food loss through improved storage, transportation, and processing technologies. This will help ensure that livestock production contributes effectively to global food security.
Enhancing Emission Tracking and Reporting
There is a need for standardized methods for collecting and reporting data on GHG emissions in agriculture. This will enable more accurate assessments and the development of targeted strategies for emission reductions.
References
Bell, D. D. (2002). Laying hens in the U.S. market: An appraisal of trends from the beginning of the 20th century to present. Poultry Science, 81(5), 485-490. https://doi.org/10.1093/ps/81.5.485
CarbonWise. (2023). Global greenhouse gas emissions by sector. Retrieved from https://carbonwise.co/global-greenhouse-gas-emissions-by-sector/
Crippa, M., Solazzo, E., Guizzardi, D., Monforti-Ferrario, F., Tubiello, F. N., Leip, A., … & Janssens-Maenhout, G. (2022). Greenhouse gas emissions from food systems: building the global food system emissions database (GFED). Earth System Science Data, 14(4), 1795-1821. https://essd.copernicus.org/articles/14/1795/2022/essd-14-1795-2022.pdf
European Environment Agency (EEA). (2023). Improving the climate impact of raw material sourcing. Retrieved from https://www.eea.europa.eu/publications/improving-the-climate-impact-of-raw-material-sourcing
Food and Agriculture Organization of the United Nations (FAO). (2021). The State of Food and Agriculture 2021: Making agrifood systems more resilient to shocks and stresses. FAO. https://openknowledge.fao.org/server/api/core/bitstreams/6e04f2b4-82fc-4740-8cd5-9b66f5335239/content
Food and Agriculture Organization of the United Nations (FAO). (2021). Food Loss and Waste Database. FAO. https://www.fao.org/platform-food-loss-waste/food-loss/introduction/en
Food and Agriculture Organization of the United Nations (FAO). (2021). Greenhouse gas emissions from agrifood systems. Retrieved from https://www.fao.org/platform-food-loss-waste/food-loss/introduction/en
Goldewijk, K. K., & Verburg, P. H. (2013). Per-capita estimations of long-term historical land use and the consequences for global change research. Global Environmental Change, 23(4), 1166-1175. https://doi.org/10.1016/j.gloenvcha.2013.04.001
Intergovernmental Panel on Climate Change (IPCC). (2023). AR6 Synthesis Report: Climate Change 2023. IPCC. https://www.ipcc.ch/report/ar6/syr/
Kusuma, A. B., Laga, W. R., & Purnomo, H. (2022). Climate Change and Livestock Farming: Strategies for Mitigation and Adaptation. MDPI, 12(10), 1554. https://www.mdpi.com/2077-0472/12/10/1554
Matthews, D. (2023). Chicken, meat, and the future of global food: Forecasts and predictions for beef, pork, and more. Vox. https://www.vox.com/future-perfect/2023/8/4/23818952/chicken-meat-forecast-predictions-beef-pork-oecd-fao?mc_cid=d1a37e53b6&mc_eid=1b5c5e908a
Our World in Data. (2020). Milk yields per animal. Retrieved from https://ourworldindata.org/grapher/milk-yields-per-animal
Our World in Data. (2023). Grazing land use over the long-term, 1600 to 2023. Retrieved from https://ourworldindata.org/grazing-land-use-over-the-long-term
Ritchie, H., & Roser, M. (2020). Food greenhouse gas emissions. Our World in Data. https://ourworldindata.org/food-ghg-emissions
Roche, J. R., Friggens, N. C., Kay, J. K., Fisher, M. W., Stafford, K. J., & Berry, D. P. (2013). Invited review: Body condition score and its association with dairy cow productivity, health, and welfare. Animal Frontiers, 3(4), 23-29. https://doi.org/10.2527/af.2013-0032
Sharma, V. P., & Gulati, A. (2020). Changes in Herd Composition a Key to Indian Dairy Production. United States Department of Agriculture (USDA) Economic Research Service. https://www.ers.usda.gov/publications/pub-details/?pubid=99794
The Last Glaciers. (2023). Decarbonizing Food and Agriculture. Retrieved from https://thelastglaciers.com/decarbonising-food-and-agriculture/
Thoma, G., Jolliet, O., & Wang, Y. (2016). National Pork Board. (2016). Greenhouse gas emissions and the potential for mitigation from the pork industry in the U.S. Retrieved from https://www.porkcheckoff.org/wp-content/uploads/2021/05/16-214-THOMA-final-rpt.pdf
Thornton, P. K., & Herrero, M. (2015). Impacts of climate change on the livestock food supply chain; a review of the evidence. Frontiers in Veterinary Science, 2, 93. https://www.ncbi.nlm.nih.gov/pmc/articles/PMC4686767/
USDA – National Agricultural Statistics Service. (n.d.). Trends in U.S. Agriculture – Broiler Industry. U.S. Department of Agriculture. Retrieved from https://www.nass.usda.gov/Publications/Trends_in_U.S._Agriculture/Broiler_Industry/
Zuidhof, M. J., Schneider, B. L., Carney, V. L., Korver, D. R., & Robinson, F. E. (2014). Evolution of the modern broiler and feed efficiency. Annual Review of Animal Biosciences, 2(1), 47-71. https://doi.org/10.1146/annurev-animal-022513-114132